



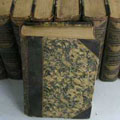
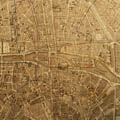


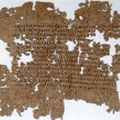
THE eighteenth-century philosopher made great strides in his studies of the physical properties of matter and the application of these properties in mechanics, as the steam-engine, the balloon, the optic telegraph, the spinning-jenny, the cotton-gin, the chronometer, the perfected compass, the Leyden jar, the lightning-rod, and a host of minor inventions testify. In a speculative way he had thought out more or less tenable conceptions as to the ultimate nature of matter, as witness the theories of Leibnitz and Boscovich and Davy, to which we may recur. But he had not as yet conceived the notion of a distinction between matter and energy, which is so fundamental to the physics of a later epoch. He did not speak of heat, light, electricity, as forms of energy or "force"; he conceived them as subtile forms of matter—as highly attenuated yet tangible fluids, subject to gravitation and chemical attraction; though he had learned to measure none of them but heat with accuracy, and this one he could test only within narrow limits until late in the century, when Josiah Wedgwood, the famous potter, taught him to gauge the highest temperatures with the clay pyrometer.
He spoke of the matter of heat as being the most universally distributed fluid in nature; as entering in some degree into the composition of nearly all other substances; as being sometimes liquid, sometimes condensed or solid, and as having weight that could be detected with the balance. Following Newton, he spoke of light as a "corpuscular emanation" or fluid, composed of shining particles which possibly are transmutable into particles of heat, and which enter into chemical combination with the particles of other forms of matter. Electricity he considered a still more subtile kind of matter-perhaps an attenuated form of light. Magnetism, "vital fluid," and by some even a "gravic fluid," and a fluid of sound were placed in the same scale; and, taken together, all these supposed subtile forms of matter were classed as "imponderables."
This view of the nature of the "imponderables" was in some measure a retrogression, for many seventeenth- century philosophers, notably Hooke and Huygens and Boyle, had held more correct views; but the materialistic conception accorded so well with the eighteenth- century tendencies of thought that only here and there a philosopher like Euler called it in question, until well on towards the close of the century. Current speech referred to the materiality of the "imponderables " unquestioningly. Students of meteorology—a science that was just dawning—explained atmospheric phenomena on the supposition that heat, the heaviest imponderable, predominated in the lower atmosphere, and that light, electricity, and magnetism prevailed in successively higher strata. And Lavoisier, the most philosophical chemist of the century, retained heat and light on a par with oxygen, hydrogen, iron, and the rest, in his list of elementary substances.
COUNT RUMFORD AND THE VIBRATORY THEORY OF HEAT
But just at the close of the century the confidence in the status of the imponderables was rudely shaken in the minds of philosophers by the revival of the old idea of Fra Paolo and Bacon and Boyle, that heat, at any rate, is not a material fluid, but merely a mode of motion or vibration among the particles of "ponderable" matter. The new champion of the old doctrine as to the nature of heat was a very distinguished philosopher and diplomatist of the time, who, it may be worth recalling, was an American. He was a sadly expatriated American, it is true, as his name, given all the official appendages, will amply testify; but he had been born and reared in a Massachusetts village none the less, and he seems always to have retained a kindly interest in the land of his nativity, even though he lived abroad in the service of other powers during all the later years of his life, and was knighted by England, ennobled by Bavaria, and honored by the most distinguished scientific bodies of Europe. The American, then, who championed the vibratory theory of heat, in opposition to all current opinion, in this closing era of the eighteenth century, was Lieutenant-General Sir Benjamin Thompson, Count Rumford, F.R.S.
Rumford showed that heat may be produced in indefinite quantities by friction of bodies that do not themselves lose any appreciable matter in the process, and claimed that this proves the immateriality of heat. Later on he added force to the argument by proving, in refutation of the experiments of Bowditch, that no body either gains or loses weight in virtue of being heated or cooled. He thought he had proved that heat is only a form of motion.
His experiment for producing indefinite quantities of heat by friction is recorded by him in his paper entitled, "Inquiry Concerning the Source of Heat Excited by Friction."
"Being engaged, lately, in superintending the boring of cannon in the workshops of the military arsenal at Munich," he says, "I was struck with the very considerable degree of heat which a brass gun acquires in a short time in being bored; and with the still more intense heat (much greater than that of boiling water, as I found by experiment) of the metallic chips separated from it by the borer.
"Taking a cannon (a brass six-pounder), cast solid, and rough, as it came from the foundry, and fixing it horizontally in a machine used for boring, and at the same time finishing the outside of the cannon by turning, I caused its extremity to be cut off; and by turning down the metal in that part, a solid cylinder was formed, 7 3/4 inches in diameter and 9 8/10 inches long; which, when finished, remained joined to the rest of the metal (that which, properly speaking, constituted the cannon) by a small cylindrical neck, only 2 1/5 inches in diameter and 3 8/10 inches long.
"This short cylinder, which was supported in its horizontal position, and turned round its axis by means of the neck by which it remained united to the cannon, was now bored with the horizontal borer used in boring cannon.
"This cylinder being designed for the express purpose of generating heat by friction, by having a blunt borer forced against its solid bottom at the same time that it should be turned round its axis by the force of horses, in order that the heat accumulated in the cylinder might from time to time be measured, a small, round hole 0.37 of an inch only in diameter and 4.2 inches in depth, for the purpose of introducing a small cylindrical mercurial thermometer, was made in it, on one side, in a direction perpendicular to the axis of the cylinder, and ending in the middle of the solid part of the metal which formed the bottom of the bore.
"At the beginning of the experiment, the temperature of the air in the shade, as also in the cylinder, was just sixty degrees Fahrenheit. At the end of thirty minutes, when the cylinder had made 960 revolutions about its axis, the horses being stopped, a cylindrical mercury thermometer, whose bulb was 32/100 of an inch in diameter and 3 1/4 inches in length, was introduced into the hole made to receive it in the side of the cylinder, when the mercury rose almost instantly to one hundred and thirty degrees.
"In order, by one decisive experiment, to determine whether the air of the atmosphere had any part or not in the generation of the heat, I contrived to repeat the experiment under circumstances in which it was evidently impossible for it to produce any effect whatever. By means of a piston exactly fitted to the mouth of the bore of the cylinder, through the middle of which piston the square iron bar, to the end of which the blunt steel borer was fixed, passed in a square hole made perfectly air-tight, the excess of the external air, to the inside of the bore of the cylinder, was effectually prevented. I did not find, however, by this experiment that the exclusion of the air diminished in the smallest degree the quantity of heat excited by the friction.
"There still remained one doubt, which, though it appeared to me to be so slight as hardly to deserve any attention, I was, however, desirous to remove. The piston which choked the mouth of the bore of the cylinder, in order that it might be air-tight, was fitted into it with so much nicety, by means of its collars of leather, and pressed against it with so much force, that, notwithstanding its being oiled, it occasioned a considerable degree of friction when the hollow cylinder was turned round its axis. Was not the heat produced, or at least some part of it, occasioned by this friction of the piston? and, as the external air had free access to the extremity of the bore, where it came into contact with the piston, is it not possible that this air may have had some share in the generation of the heat produced?
"A quadrangular oblong deal box, water-tight, being provided with holes or slits in the middle of each of its ends, just large enough to receive, the one the square iron rod to the end of which the blunt steel borer was fastened, the other the small cylindrical neck which joined the hollow cylinder to the cannon; when this box (which was occasionally closed above by a wooden cover or lid moving on hinges) was put into its place— that is to say, when, by means of the two vertical opening or slits in its two ends, the box was fixed to the machinery in such a manner that its bottom being in the plane of the horizon, its axis coincided with the axis of the hollow metallic cylinder, it is evident, from the description, that the hollow, metallic cylinder would occupy the middle of the box, without touching it on either side; and that, on pouring water into the box and filling it to the brim, the cylinder would be completely covered and surrounded on every side by that fluid. And, further, as the box was held fast by the strong, square iron rod which passed in a square hole in the centre of one of its ends, while the round or cylindrical neck which joined the hollow cylinder to the end of the cannon could turn round freely on its axis in the round hole in the centre of the other end of it, it is evident that the machinery could be put in motion without the least danger of forcing the box out of its place, throwing the water out of it, or deranging any part of the apparatus."
Everything being thus ready, the box was filled with cold water, having been made water-tight by means of leather collars, and the machinery put in motion. "The result of this beautiful experiment," says Rumford, "was very striking, and the pleasure it afforded me amply repaid me for all the trouble I had had in contriving and arranging the complicated machinery used in making it. The cylinder, revolving at the rate of thirty-two times in a minute, had been in motion but a short time when I perceived, by putting my hand into the water and touching the outside of the cylinder, that heat was generated, and it was not long before the water which surrounded the cylinder began to be sensibly warm.
"At the end of one hour I found, by plunging a thermometer into the box, . . . that its temperature had been raised no less than forty-seven degrees Fahrenheit, being now one hundred and seven degrees Fahrenheit. ... One hour and thirty minutes after the machinery had been put in motion the heat of the water in the box was one hundred and forty-two degrees. At the end of two hours ... it was raised to one hundred and seventy-eight degrees; and at two hours and thirty minutes it ACTUALLY BOILED!
"It would be difficult to describe the surprise and astonishment expressed in the countenances of the bystanders on seeing so large a quantity of cold water heated, and actually made to boil, without any fire. Though there was, in fact, nothing that could justly be considered as a surprise in this event, yet I acknowledge fairly that it afforded me a degree of childish pleasure which, were I ambitious of the reputation of a GRAVE PHILOSOPHER, I ought most certainly rather to hide than to discover...."
Having thus dwelt in detail on these experiments, Rumford comes now to the all-important discussion as to the significance of them—the subject that had been the source of so much speculation among the philosophers— the question as to what heat really is, and if there really is any such thing (as many believed) as an igneous fluid, or a something called caloric.
"From whence came this heat which was continually given off in this manner, in the foregoing experiments?" asks Rumford. "Was it furnished by the small particles of metal detached from the larger solid masses on their being rubbed together? This, as we have already seen, could not possibly have been the case.
"Was it furnished by the air? This could not have been the case; for, in three of the experiments, the machinery being kept immersed in water, the access of the air of the atmosphere was completely prevented.
"Was it furnished by the water which surrounded the machinery? That this could not have been the case is evident: first, because this water was continually RECEIVING heat from the machinery, and could not, at the same time, be GIVING TO and RECEIVING HEAT FROM the same body; and, secondly, because there was no chemical decomposition of any part of this water. Had any such decomposition taken place (which, indeed, could not reasonably have been expected), one of its component elastic fluids (most probably hydrogen) must, at the same time, have been set at liberty, and, in making its escape into the atmosphere, would have been detected; but, though I frequently examined the water to see if any air-bubbles rose up through it, and had even made preparations for catching them if they should appear, I could perceive none; nor was there any sign of decomposition of any kind whatever, or other chemical process, going on in the water.
"Is it possible that the heat could have been supplied by means of the iron bar to the end of which the blunt steel borer was fixed? Or by the small neck of gun-metal by which the hollow cylinder was united to the cannon? These suppositions seem more improbable even than either of the before-mentioned; for heat was continually going off, or OUT OF THE MACHINERY, by both these passages during the whole time the experiment lasted.
"And in reasoning on this subject we must not forget to consider that most remarkable circumstance, that the source of the heat generated by friction in these experiments appeared evidently to be INEXHAUSTIBLE.
"It is hardly necessary to add that anything which any INSULATED body, or system of bodies, can continue to furnish WITHOUT LIMITATION cannot possibly be a MATERIAL substance; and it appears to me to be extremely difficult, if not quite impossible, to form any distinct idea of anything capable of being excited and communicated, in the manner the heat was excited and communicated in these experiments, except in MOTION."[1]
THOMAS YOUNG AND THE WAVE THEORY OF LIGHT
But contemporary judgment, while it listened respectfully to Rumford, was little minded to accept his verdict. The cherished beliefs of a generation are not to be put down with a single blow. Where many minds have a similar drift, however, the first blow may precipitate a general conflict; and so it was here. Young Humphry Davy had duplicated Rumford's experiments, and reached similar conclusions; and soon others fell into line. Then, in 1800, Dr. Thomas Young— "Phenomenon Young" they called him at Cambridge, because he was reputed to know everything—took up the cudgels for the vibratory theory of light, and it began to be clear that the two "imponderables," heat and light, must stand or fall together; but no one as yet made a claim against the fluidity of electricity.
Before we take up the details of the assault made by Young upon the old doctrine of the materiality of light, we must pause to consider the personality of Young himself. For it chanced that this Quaker physician was one of those prodigies who come but few times in a century, and the full list of whom in the records of history could be told on one's thumbs and fingers. His biographers tell us things about him that read like the most patent fairy-tales. As a mere infant in arms he had been able to read fluently. Before his fourth birthday came he had read the Bible twice through, as well as Watts's Hymns—poor child!—and when seven or eight he had shown a propensity to absorb languages much as other children absorb nursery tattle and Mother Goose rhymes. When he was fourteen, a young lady visiting the household of his tutor patronized the pretty boy by asking to see a specimen of his penmanship. The pretty boy complied readily enough, and mildly rebuked his interrogator by rapidly writing some sentences for her in fourteen languages, including such as, Arabian, Persian, and Ethiopic.
Meantime languages had been but an incident in the education of the lad. He seems to have entered every available field of thought—mathematics, physics, botany, literature, music, painting, languages, philosophy, archaeology, and so on to tiresome lengths—and once he had entered any field he seldom turned aside until he had reached the confines of the subject as then known and added something new from the recesses of his own genius. He was as versatile as Priestley, as profound as Newton himself. He had the range of a mere dilettante, but everywhere the full grasp of the master. He took early for his motto the saying that what one man has done, another man may do. Granting that the other man has the brain of a Thomas Young, it is a true motto.
Such, then, was the young Quaker who came to London to follow out the humdrum life of a practitioner of medicine in the year 1801. But incidentally the young physician was prevailed upon to occupy the interims of early practice by fulfilling the duties of the chair of Natural Philosophy at the Royal Institution, which Count Rumford had founded, and of which Davy was then Professor of Chemistry—the institution whose glories have been perpetuated by such names as Faraday and Tyndall, and which the Briton of to-day speaks of as the "Pantheon of Science." Here it was that Thomas Young made those studies which have insured him a niche in the temple of fame not far removed from that of Isaac Newton.
As early as 1793, when he was only twenty, Young had begun to Communicate papers to the Royal Society of London, which were adjudged worthy to be printed in full in the Philosophical Transactions; so it is not strange that he should have been asked to deliver the Bakerian lecture before that learned body the very first year after he came to London. The lecture was delivered November 12, 1801. Its subject was "The Theory of Light and Colors," and its reading marks an epoch in physical science; for here was brought forward for the first time convincing proof of that undulatory theory of light with which every student of modern physics is familiar—the theory which holds that light is not a corporeal entity, but a mere pulsation in the substance of an all-pervading ether, just as sound is a pulsation in the air, or in liquids or solids.
Young had, indeed, advocated this theory at an earlier date, but it was not until 1801 that he hit upon the idea which enabled him to bring it to anything approaching a demonstration. It was while pondering over the familiar but puzzling phenomena of colored rings into which white light is broken when reflected from thin films—Newton's rings, so called—that an explanation occurred to him which at once put the entire undulatory theory on a new footing. With that sagacity of insight which we call genius, he saw of a sudden that the phenomena could be explained by supposing that when rays of light fall on a thin glass, part of the rays being reflected from the upper surface, other rays, reflected from the lower surface, might be so retarded in their course through the glass that the two sets would interfere with one another, the forward pulsation of one ray corresponding to the backward pulsation of another, thus quite neutralizing the effect. Some of the component pulsations of the light being thus effaced by mutual interference, the remaining rays would no longer give the optical effect of white light; hence the puzzling colors.
Here is Young's exposition of the subject:
Of the Colors of Thin Plates
"When a beam of light falls upon two refracting surfaces, the partial reflections coincide perfectly in direction; and in this case the interval of retardation taken between the surfaces is to their radius as twice the cosine of the angle of refraction to the radius.
"Let the medium between the surfaces be rarer than the surrounding mediums; then the impulse reflected at the second surface, meeting a subsequent undulation at the first, will render the particles of the rarer medium capable of wholly stopping the motion of the denser and destroying the reflection, while they themselves will be more strongly propelled than if they had been at rest, and the transmitted light will be increased. So that the colors by reflection will be destroyed, and those by transmission rendered more vivid, when the double thickness or intervals of retardation are any multiples of the whole breadth of the undulations; and at intermediate thicknesses the effects will be reversed according to the Newtonian observation.
"If the same proportions be found to hold good with respect to thin plates of a denser medium, which is, indeed, not improbable, it will be necessary to adopt the connected demonstrations of Prop. IV., but, at any rate, if a thin plate be interposed between a rarer and a denser medium, the colors by reflection and transmission may be expected to change places.
Of the Colors of Thick Plates
"When a beam of light passes through a refracting surface, especially if imperfectly polished, a portion of it is irregularly scattered, and makes the surface visible in all directions, but most conspicuously in directions not far distant from that of the light itself; and if a reflecting surface be placed parallel to the refracting surface, this scattered light, as well as the principal beam, will be reflected, and there will be also a new dissipation of light, at the return of the beam through the refracting surface. These two portions of scattered light will coincide in direction; and if the surfaces be of such a form as to collect the similar effects, will exhibit rings of colors. The interval of retardation is here the difference between the paths of the principal beam and of the scattered light between the two surfaces; of course, wherever the inclination of the scattered light is equal to that of the beam, although in different planes, the interval will vanish and all the undulations will conspire. At other inclinations, the interval will be the difference of the secants from the secant of the inclination, or angle of refraction of the principal beam. From these causes, all the colors of concave mirrors observed by Newton and others are necessary consequences; and it appears that their production, though somewhat similar, is by no means as Newton imagined, identical with the production of thin plates."[2]
By following up this clew with mathematical precision, measuring the exact thickness of the plate and the space between the different rings of color, Young was able to show mathematically what must be the length of pulsation for each of the different colors of the spectrum. He estimated that the undulations of red light, at the extreme lower end of the visible spectrum, must number about thirty-seven thousand six hundred and forty to the inch, and pass any given spot at a rate of four hundred and sixty-three millions of millions of undulations in a second, while the extreme violet numbers fifty-nine thousand seven hundred and fifty undulations to the inch, or seven hundred and thirty-five millions of millions to the second.
The Colors of Striated Surfaces
Young similarly examined the colors that are produced by scratches on a smooth surface, in particular testing the light from "Mr. Coventry's exquisite micrometers," which consist of lines scratched on glass at measured intervals. These microscopic tests brought the same results as the other experiments. The colors were produced at certain definite and measurable angles, and the theory of interference of undulations explained them perfectly, while, as Young affirmed with confidence, no other hypothesis hitherto advanced would explain them at all. Here are his words:
"Let there be in a given plane two reflecting points very near each other, and let the plane be so situated that the reflected image of a luminous object seen in it may appear to coincide with the points; then it is obvious that the length of the incident and reflected ray, taken together, is equal with respect to both points, considering them as capable of reflecting in all directions. Let one of the points be now depressed below the given plane; then the whole path of the light reflected from it will be lengthened by a line which is to the depression of the point as twice the cosine of incidence to the radius.
"If, therefore, equal undulations of given dimensions be reflected from two points, situated near enough to appear to the eye but as one, whenever this line is equal to half the breadth of a whole undulation the reflection from the depressed point will so interfere with the reflection from the fixed point that the progressive motion of the one will coincide with the retrograde motion of the other, and they will both be destroyed; but when this line is equal to the whole breadth of an undulation, the effect will be doubled, and when to a breadth and a half, again destroyed; and thus for a considerable number of alternations, and if the reflected undulations be of a different kind, they will be variously affected, according to their proportions to the various length of the line which is the difference between the lengths of their two paths, and which may be denominated the interval of a retardation.
"In order that the effect may be the more perceptible, a number of pairs of points must be united into two parallel lines; and if several such pairs of lines be placed near each other, they will facilitate the observation. If one of the lines be made to revolve round the other as an axis, the depression below the given plane will be as the sine of the inclination; and while the eye and the luminous object remain fixed the difference of the length of the paths will vary as this sine.
"The best subjects for the experiment are Mr. Coventry's exquisite micrometers; such of them as consist of parallel lines drawn on glass, at a distance of one- five-hundredth of an inch, are the most convenient. Each of these lines appears under a microscope to consist of two or more finer lines, exactly parallel, and at a distance of somewhat more than a twentieth more than the adjacent lines. I placed one of these so as to reflect the sun's light at an angle of forty-five degrees, and fixed it in such a manner that while it revolved round one of the lines as an axis, I could measure its angular motion; I found that the longest red color occurred at the inclination 10 1/4 degrees, 20 3/4 degrees, 32 degrees, and 45 degrees; of which the sines are as the numbers 1, 2, 3, and 4. At all other angles also, when the sun's light was reflected from the surface, the color vanished with the inclination, and was equal at equal inclinations on either side.
This experiment affords a very strong confirmation of the theory. It is impossible to deduce any explanation of it from any hypothesis hitherto advanced; and I believe it would be difficult to invent any other that would account for it. There is a striking analogy between this separation of colors and the production of a musical note by successive echoes from equidistant iron palisades, which I have found to correspond pretty accurately with the known velocity of sound and the distances of the surfaces.
"It is not improbable that the colors of the integuments of some insects, and of some other natural bodies, exhibiting in different lights the most beautiful versatility, may be found to be of this description, and not to be derived from thin plates. In some cases a single scratch or furrow may produce similar effects, by the reflection of its opposite edges."[3]
This doctrine of interference of undulations was the absolutely novel part of Young's theory. The all- compassing genius of Robert Hooke had, indeed, very nearly apprehended it more than a century before, as Young himself points out, but no one else bad so much as vaguely conceived it; and even with the sagacious Hooke it was only a happy guess, never distinctly outlined in his own mind, and utterly ignored by all others. Young did not know of Hooke's guess until he himself had fully formulated the theory, but he hastened then to give his predecessor all the credit that could possibly be adjudged his due by the most disinterested observer. To Hooke's contemporary, Huygens, who was the originator of the general doctrine of undulation as the explanation of light, Young renders full justice also. For himself he claims only the merit of having demonstrated the theory which these and a few others of his predecessors had advocated without full proof.
The following year Dr. Young detailed before the Royal Society other experiments, which threw additional light on the doctrine of interference; and in 1803 he cited still others, which, he affirmed, brought the doctrine to complete demonstration. In applying this demonstration to the general theory of light, he made the striking suggestion that "the luminiferous ether pervades the substance of all material bodies with little or no resistance, as freely, perhaps, as the wind passes through a grove of trees." He asserted his belief also that the chemical rays which Ritter had discovered beyond the violet end of the visible spectrum are but still more rapid undulations of the same character as those which produce light. In his earlier lecture he had affirmed a like affinity between the light rays and the rays of radiant heat which Herschel detected below the red end of the spectrum, suggesting that "light differs from heat only in the frequency of its undulations or vibrations—those undulations which are within certain limits with respect to frequency affecting the optic nerve and constituting light, and those which are slower and probably stronger constituting heat only." From the very outset he had recognized the affinity between sound and light; indeed, it had been this affinity that led him on to an appreciation of the undulatory theory of light.
But while all these affinities seemed so clear to the great co-ordinating brain of Young, they made no such impression on the minds of his contemporaries. The immateriality of light had been substantially demonstrated, but practically no one save its author accepted the demonstration. Newton's doctrine of the emission of corpuscles was too firmly rooted to be readily dislodged, and Dr. Young had too many other interests to continue the assault unceasingly. He occasionally wrote something touching on his theory, mostly papers contributed to the Quarterly Review and similar periodicals, anonymously or under pseudonym, for he had conceived the notion that too great conspicuousness in fields outside of medicine would injure his practice as a physician. His views regarding light (including the original papers from the Philosophical Transactions of the Royal Society) were again given publicity in full in his celebrated volume on natural philosophy, consisting in part of his lectures before the Royal Institution, published in 1807; but even then they failed to bring conviction to the philosophic world. Indeed, they did not even arouse a controversial spirit, as his first papers had done.
ARAGO AND FRESNEL CHAMPION THE WAVE THEORY
So it chanced that when, in 1815, a young French military engineer, named Augustin Jean Fresnel, returning from the Napoleonic wars, became interested in the phenomena of light, and made some experiments concerning diffraction which seemed to him to controvert the accepted notions of the materiality of light, he was quite unaware that his experiments had been anticipated by a philosopher across the Channel. He communicated his experiments and results to the French Institute, supposing them to be absolutely novel. That body referred them to a committee, of which, as good fortune would have it, the dominating member was Dominique Francois Arago, a man as versatile as Young himself, and hardly less profound, if perhaps not quite so original. Arago at once recognized the merit of Fresnel's work, and soon became a convert to the theory. He told Fresnel that Young had anticipated him as regards the general theory, but that much remained to be done, and he offered to associate himself with Fresnel in prosecuting the investigation. Fresnel was not a little dashed to learn that his original ideas had been worked out by another while he was a lad, but he bowed gracefully to the situation and went ahead with unabated zeal.
The championship of Arago insured the undulatory theory a hearing before the French Institute, but by no means sufficed to bring about its general acceptance. On the contrary, a bitter feud ensued, in which Arago was opposed by the "Jupiter Olympus of the Academy," Laplace, by the only less famous Poisson, and by the younger but hardly less able Biot. So bitterly raged the feud that a life-long friendship between Arago and Biot was ruptured forever. The opposition managed to delay the publication of Fresnel's papers, but Arago continued to fight with his customary enthusiasm and pertinacity, and at last, in 1823, the Academy yielded, and voted Fresnel into its ranks, thus implicitly admitting the value of his work.
It is a humiliating thought that such controversies as this must mar the progress of scientific truth; but fortunately the story of the introduction of the undulatory theory has a more pleasant side. Three men, great both in character and in intellect, were concerned in pressing its claims—Young, Fresnel, and Arago—and the relations of these men form a picture unmarred by any of those petty jealousies that so often dim the lustre of great names. Fresnel freely acknowledged Young's priority so soon as his attention was called to it; and Young applauded the work of the Frenchman, and aided with his counsel in the application of the undulatory theory to the problems of polarization of light, which still demanded explanation, and which Fresnel's fertility of experimental resource and profundity of mathematical insight sufficed in the end to conquer.
After Fresnel's admission to the Institute in 1823 the opposition weakened, and gradually the philosophers came to realize the merits of a theory which Young had vainly called to their attention a full quarter- century before. Now, thanks largely to Arago, both Young and Fresnel received their full meed of appreciation. Fresnel was given the Rumford medal of the Royal Society of England in 1825, and chosen one of the foreign members of the society two years later, while Young in turn was elected one of the eight foreign members of the French Academy. As a fitting culmination of the chapter of felicities between the three friends, it fell to the lot of Young, as Foreign Secretary of the Royal Society, to notify Fresnel of the honors shown him by England's representative body of scientists; while Arago, as Perpetual Secretary of the French Institute, conveyed to Young in the same year the notification that he had been similarly honored by the savants of France.
A few months later Fresnel was dead, and Young survived him only two years. Both died prematurely, but their great work was done, and the world will remember always and link together these two names in connection with a theory which in its implications and importance ranks little below the theory of universal gravitation.